I’m sitting in my hotel room on the morning after the 2017 LPSC Meeting, trying process all the science that washed over me in the past week. And it was a lot, as you can see by checking out the twitter hashtag #lpsc2017.
From the migration of Martian dune ripples to the global seas on Enceladus, there’s no way for me to do it all justice in one blog post. So instead, I’ll talk about one of the talks that stood out most for me.
On the last day of the conference, Dr. Colin Dundas of USGS gave a mic-droppingly good talk in which he argued very convincingly that Mars’ recurring slope lineae are NOT the result of flowing water. This is a big deal for folks who study Mars but might sound a little arcane for non-Martians.
The animated image above consists of several pictures taken by the HiRISE camera onboard the Mars Reconnaissance Orbiter over several months. The very pronounced dark streaks extending out from the cliff face – those are the recurring slope lineae or RSLs. In many locations on Mars, they recur from one year to the next; they always show up on sloped surfaces; and they are long lines. Hence recurring slope lineae.
Under the current conditions at Mars’ surface, liquid water is not stable over long times, but these dark streaks sure look like small amounts of water running out over the surface, which got people very excited when they were first discovered.
In fact, just a few years ago, some scientists analyzed the spectra of some RSLs and argued they were highly salty brine flows. The salt is important because, even though pure water can’t exist on the surface of Mars, large amounts of salt can stabilize the water, at least for a little while.
This was a huge result, not just because it meant RSLs were small amounts of running water on Mars but because it raised the possibility of much larger sub-surface reservoirs of water. And where there’s lots of water, there could be life.
Well, Dundas’s talk throws all that into doubt. By analyzing the angles of the slopes on which the RSLs occur, Dundas showed that they almost every one of them ended on a slope of about 30 degrees.
Now, if RSLs are flowing water, they should keep flowing, even on small slopes. But if they are some sort of dry granular flow instead, you would expect them to stop once they reached the angle of repose, which is about 30 degrees.
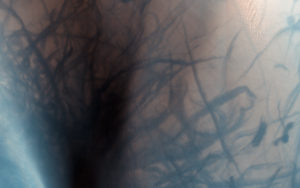
Dark streaks on the sides of Martian dunes seen by HiRISE. These are NOT due to liquid water but resemble in many ways the RSLs.
To bolster his argument, Dundas showed several examples of dry granular flows on Mars that exhibited many of the same properties as RSLs – recurring dark streaks, running along sloped surfaces.
Continued work will either corroborate Dundas’s striking result or circumvent it, and given the number of folks lined up to talk with him after his talk, I’m sure the fans of liquid-flow RSLs will work hard to counter his arguments. And that’s how science progresses – one falsified hypothesis at a time.
Not quite as splashy, I also gave a presentation on our work trying to de-bias dust devil surveys. I’ve posted the presentation below.
All in all, my first LPSC was a great mix of science, warm weather, and warm friends.