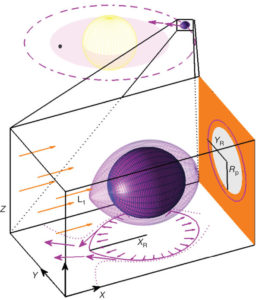
(top) WASP-12 b’s orbit. (bottom) The Roche lobe around WASP-12 b.
WASP-12 b is a planet in crisis.
One of the hottest of the hot Jupiters, the gas giant circles its Sun-like host star in a blistering 1.09-day orbit, giving its an atmosphere hot enough to vaporize rubies.
In fact, WASP-12 b is so close to its star that it very nearly fills its Roche lobe, the teardrop-shaped region inside of which material is bound to the planet. Anything on the other side of the Roche lobe falls into the gravitational clutches of the host star and can either tumble into the star or leave the system altogether.
Because WASP-12 b’s atmosphere is so hot, it is very puffy and extended, and a study from several years ago pointed out that some of the atmosphere can probably spill over the Roche lobe and escape the planet. Indeed, several groups have seen indications of outflow from WASP-12 b, meaning the planet is falling apart in front of our eyes.
Knowing what happens to the gas after escaping the planet is important for understanding the fate of the planet. If the gas goes into orbit around the star, forming an accretion disk, the planet might have billions of years before it’s destroyed. On the other hand, if the gas quickly escapes from the system or is otherwise prevented from forming a disk, the star’s gravity could rip the planet apart in an astronomical blink-of-an-eye.
A recent study from Alex Debrecht and colleagues from University of Rochester Physics and Astronomy explored what happens to gas escaping WASP-12 b. For their study, they constructed hydrodynamic models using the AstroBEAR code and found that a substantial torus of hot gas could build up around the host star in about a decade, potentially enough gas to explain the observations showing some kind of spectral absorption from the system. Such tori may commonly form in systems with ultra-hot Jupiters, so this study is probably relevant to lots of exotic exoplanets.
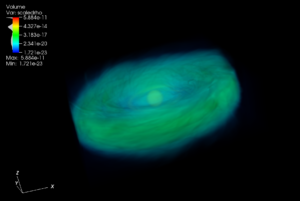
Three-dimensional simulation of gas torus in the WASP-12 system.
As interesting as these results are, though, they leave some important issues unaddressed. For instance, Debrecht and colleagues didn’t seem to include any accretion onto the host star, which might happen proceed at a fairly high rate. And the balance between outflow from the planet and accretion onto the star will go a long way to determining the amount of material in the accretion disk.
And knowing the amount of material in the disk on orbits interior and exterior to the planet’s orbit is critical for understanding the fate of WASP-12 b and other similar ultra-hot Jupiters. The accretion disk can gravitationally tug on the planet – material interior can push the planet out, while material exterior can push the planet in, potentially dooming the planet to rapid disruption.
But as is true for even the most seminal scientific work, more research is needed.