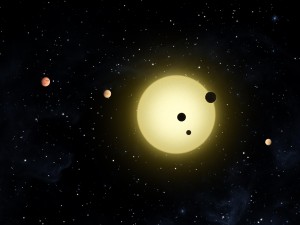
Three planets in the Kepler-11 system as they simultaneously transit their star as imagined by by a NASA artist (Image credit: NASA). From http://ciera.northwestern.edu/Research/highlights/research_highlights.php#ForeignWorlds.
Great finish to the meeting, and thankfully no big disasters at my special session.
Lots of excellent talks, but the talk that stood out for me was Sarah Ballard’s, in which she addressed an impressively simple but compelling question: Is there a difference between planetary systems where we’ve only found one planet and systems where we’ve found more than one?
This question is important because such a difference could point to different formation and/or evolutionary processes in these systems, and so comparison of these systems could elucidate subtle but significant aspects of planet formation.
In fact, Ballard did find the two types of planetary system are different, and that, for some reason, about half of M-dwarf stars that host planets have only one.
She also found modest but intriguing differences in the stars that host single planet: their features suggest they may be older than stars with multi-planet systems. Does that mean that single-planet stars used to have multiple planets but enough time has passed that the system became dynamically unstable, leaving behind a single planet?