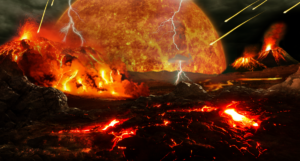
Artist’s conception of early Earth.
The Earth is about 4.5 billion years old, and it’s seen some dramatic events during that time – from the giant Moon-forming impact to the advent of fidget spinners.
The earliest phase in the Earth’s history, called the Hadean, was hellish. During long stretches, the surface was probably molten, the atmosphere was toxic, and there were no oceans. Eventually, though, the Earth transitioned to its current hospitable condition, and life got a toehold by at least 3.5 billion years ago.
But today in the Boise State Geosciences seminar, we heard about possible evidence for life going back almost a billion years earlier from Dr. Elisabeth Bell of UCLA’s Earth, Planetary, and Space Sciences Dept.
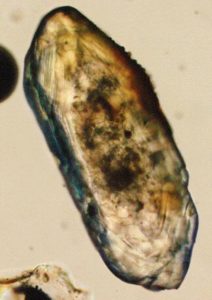
Optical microscope photograph; the length of the crystal is about 250 µm.
Dr. Bell’s work focuses on the hardiest of all mineral grains, the mighty zircons. These little rocks can be smaller than the width of a human hair, but they provide some of the strongest clues about conditions on the early Earth.
The mineral from which they’re made is very tough, and so weathering processes that usually break down other minerals barely affect zircons at all. Consequently, zircons that formed billions of years ago still retain their integrity, and, like the amber from “Jurassic Park”, often contain treasures in the form of other minerals. Dr. Bell and her team analyze these mineral time-capsules to learn what the early Earth was like.
One of the most exciting finds from her work comes from the Jack Hills geological formation in western Australia, where rocks almost as old as the Earth can be found. Inside these rocks are even older zircons, dating back to 4.1 billion years ago, which themselves have trapped small grains of graphite.
By analyzing the isotopic composition of these graphite grains, Dr. Bell has found tantalizing evidence for chemical processing of carbon that resembles biology. Although the evidence is still tentative, the results suggest life on Earth started chugging away smack in the middle of the Hadean, much earlier than has previously been believed.
Taking a step back, such a result suggests that life may get started on an Earth-like planet very quickly, which could mean that life is an almost inevitable outcome of the evolution of Earth-like planets. Given how common Earth-like planets may be, that could mean the universe may be replete with at least simple life.