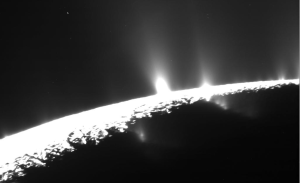
The geysers near Enceladus’ south pole. From https://en.wikipedia.org/wiki/Enceladus#/media/File:PIA19061-SaturnMoonEnceladus-CurtainNotDiscrete-Eruptions-20150506.jpg.
Saturn’s moon Enceladus has inspired fascination since Herschel found it in the late 1700s. The discovery of active cryovolcanoes geysers** on its surface by the Cassini mission in 2006 raised that fascination to a feverous intensity.
Although the source of energy powering the volcanoes geysers** was not (and is still not) understood, their implication was clear: Enceladus could have a sub-surface ocean, like his big sister Europa.
The follow-up discovery of salty particles in the geysers all but clinched the existence of a sub-surface water source. Salt in the eruptions would probably require liquid water to dissolve rocky materials to produce the salt. But it wasn’t clear whether the sub-surface source is a small pocket of water directly beneath the geysers or a more global-scale ocean.
In our journal club today, we discussed a paper that points to the existence of a global ocean. The recently published study from Peter Thomas and colleagues analyzed Enceladus’ rotation to study its internal structure. By tracking the motion of hundreds of control points on Enceladus’ surface, they found that its outer icy crust oscillates back and forth during its rotation much more than it should if the moon were solid all the way through.
Thomas and colleagues show that the large oscillations they found (called libration) require a large layer of fluid within Enceladus to lubricate the space between its outer, icy shell and its rocky interior. Otherwise, the moon would oscillate a lot less. Difficult to say exactly, but Thomas and colleagues estimate the ocean could be as thick as 30 km beneath an 20-km thick icy crust.
Just as Europa’s ocean, a sub-surface ocean in Enceladus could represent an enormous harbor for life. Even on this tiny moon, such a deep ocean is only about a tenth the size of the Earth’s oceans*.
Although Enceladus’ surface would be a tough neighborhood for life, sub-surface biota (if they ever evolved) would be protected by a thick layer of ice from the vacuum of space and interplanetary radiation. In fact, this same radiation could impinge on the surface and produce a steady supply of biologically useful oxidants, which could then trickle down into the subsurface ocean and help power the alien biosphere.
Thomas and colleagues suggest a more detailed analysis of Enceladus’ surface geology, newly inspired by their discovery, might help unravel the history of the ocean. Similar analyses of Europa’s complex tangle of surface ridges and cracks helped piece together that moon’s geological history.
Journal club attendees today included Jennifer Briggs, Hari Gopalakrishnan, Tyler Gordon, and Jacob Sabin.
*Earth’s oceans are, on average, 4 km deep, much smaller than Earth’s radius of 6,400 km. Therefore, I approximated their volume as pi*(6,400 km)^2 (4 km) ~ 500 Mkm^3. A similar calculation for Enceladus’ ocean — pi*(50 km)^2 (30 km) ~ 24 Mkm^3.
**Dr. Thomas kindly pointed out that there is some debate over the nature of the eruptions on Enceladus, and volcanoes is probably not the term to use.